Research
Our Research Areas
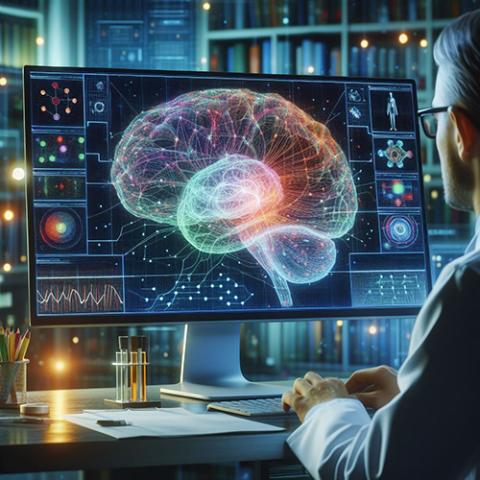
CeMSIM researchers are developing unique imaging modalities via the joint design of imaging hardware and image formation algorithms to provide new solutions with unprecedented capabilities for a wide array of biomedical applications. Researchers are active in integrating innovative imaging systems for precision medicine, pioneering quantum imaging and detection methodologies for biomedical applications, developing dedicated AI-based image formation/processing pipelines/diagnostic models and associated novel methodologies at the interface of AI and simulation-based inference.
Lead: Dr. Xavier Intes | Co-lead: Dr. Moussa Ngom
- In Vivo FRET Imaging for Targeted Drug Delivery Assessment
- Hyperspectral Wide-Field Single-Pixel Time-Resolved Optical Imaging
- Deep Learning for Enhanced 3D/4D Optical Imaging
- Hardware-Based Neural Network Accelerators for Onboard Computational Imaging
- Single Photon Generation
- Optical Mode Control in Multimode Fibers for Single Fiber Endoscopy
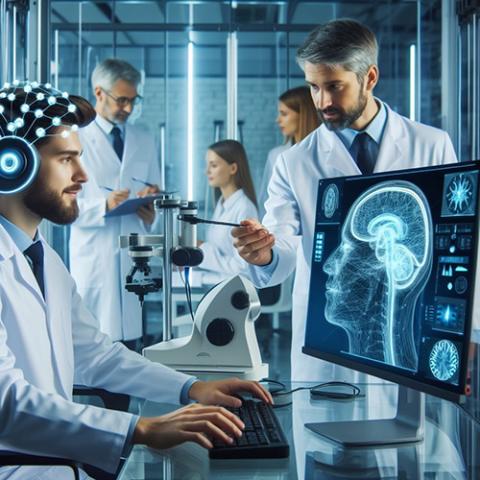
CeMSIM researchers are developing and employing novel methodologies to study the human brain in relation to performance at work and in everyday setting. These efforts leverage expertise in electrical (EEG) and optical imaging systems (fNIRS), dedicated data processing pipelines, AI-based classification and inferences, and Physics-based simulation workflows. These new methodologies are deployed in the context of high-stake professional settings such as surgical skill execution.
Lead: Dr. Xavier Intes | Co-lead: Dr. Stefan T. Radev
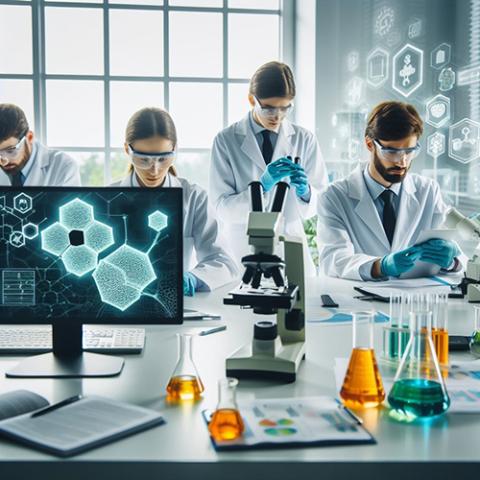
CeMSIM researchers are developing novel bioprinting and biofabrication methods to engineer precise cell cultures, functional tissue constructs, tissue systems, and in vitro models of development and disease. The integration of multiple fabrication techniques enables spatially- and compositionally-precise living, cellular constructs to be created for fundamental biologic investigations, and disease modeling. Researchers also employ a variety of novel characterization techniques, including non-destructive methods, to assess the biologic viability, morphology, and biomechanical aspects of engineered constructs during development, and in response to therapeutic treatment.
Lead: Dr. David Corr | Co-lead: Dr. Leo Wan
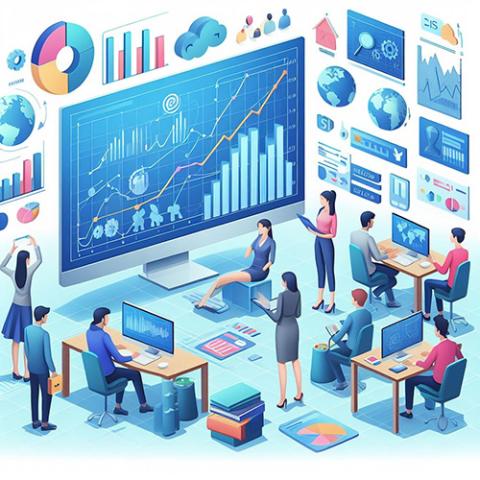
CeMSIM researchers employ a multi-disciplinary approach that combines novel theoretical and computational models, along with data-driven analytics, for biomedical modeling and simulation. Researchers are actively engaged in developing novel physics-based models and real-time algorithms and simulation technologies, and employing them in a wide range of biologically and clinically relevant applications, such as tissue development, pre-operative surgical planning, soft tissue injury and wound healing.
Lead: Dr. Rahul Rahul | Co-lead: Dr. Lucy Zhang